[EXCLUSIVE INTERVIEW BY RINNOVABILI] 100% Renewable Energy: myth or reality? According to Mark Jacobson, a professor at Stanford University and one of the world's leading energy experts, we already have 97% of the necessary technologies. And we don’t need gas, nuclear, CCS, or other false solutions
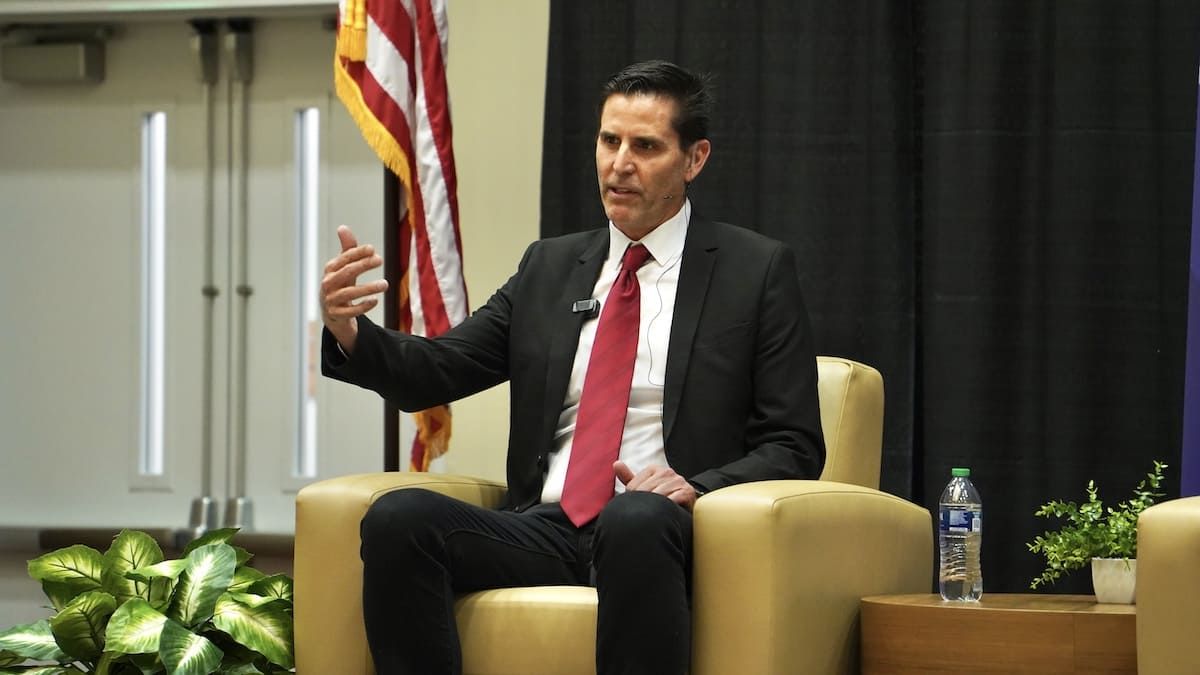
What technologies and policies do we need for a transition to 100% renewable energy?
A global transition to 100% renewable energy is already possible with existing technologies. Electrification, combined with wind, solar, and hydropower, can eliminate dependence on fossil fuels. Without requiring speculative solutions like nuclear power – including Small Nuclear Reactors, SMRs – or Carbon Capture (CCS). While simultaneously reducing costs, emissions, and environmental risks.
It’s the key message shared by Mark Jacobson in his 2023 book ‘No Miracles Needed: How Today’s Technology Can Save Our Climate and Clean Our Air’, an updated version of which is due in 2025, where the Stanford professor in civil and environmental engineering discusses which technologies are not needed but are currently being pursued.
Rinnovabili interviewed Jacobson, a leading global expert on energy and climate, about his models for global energy transitions and plans for achieving 100% renewable energy.
In your book No Miracles Needed, you emphasize that we already have all the technologies required for a transition to 100% renewable energy. What are the biggest technical challenges?
About 97% of the necessary technologies already exist. The remaining 3% mainly concern long-distance transport, such as long-distance aircraft and ships, which can be powered by hydrogen fuel cells. For buildings, we have electric heat pumps, induction cooktops, and LED lights.
A significant issue is the transmission infrastructure—zoning laws make it difficult to build new lines, and in fire-prone areas, undergrounding them is costly.
However, distributed solar energy, particularly rooftop solar, helps alleviate this challenge by reducing grid demand and enabling local energy self-sufficiency.
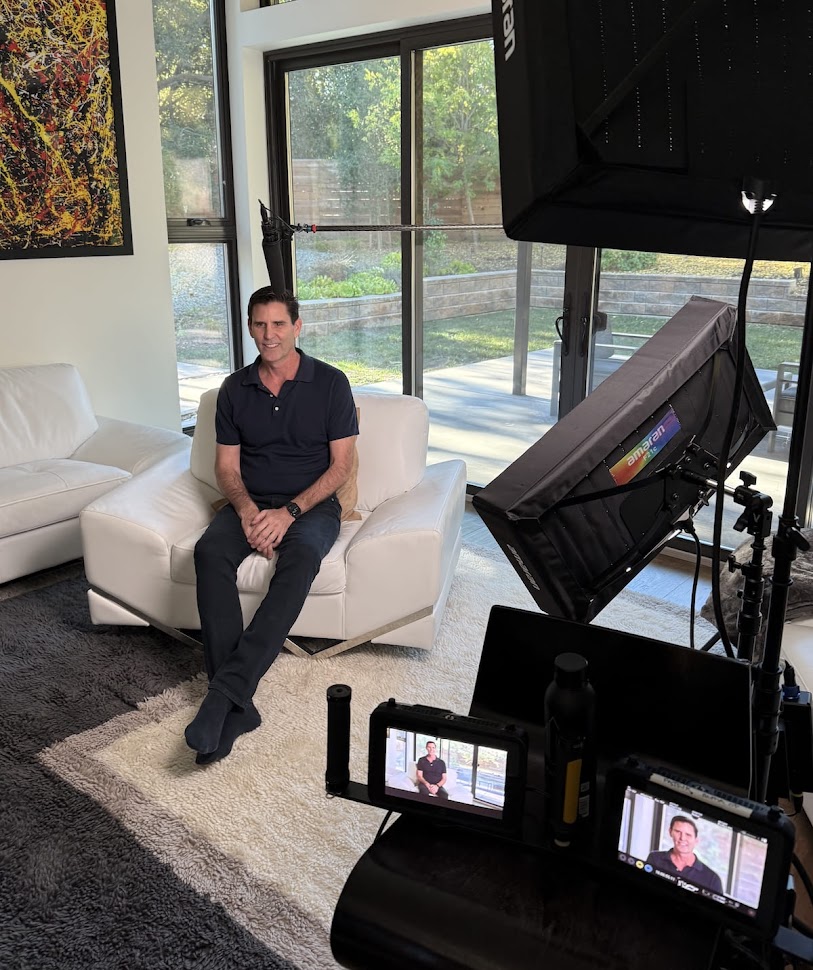
Another challenge is the expansion of energy storage systems. While battery storage is rapidly advancing, other storage solutions such as green hydrogen storage together with batteries for electricity backup and fire bricks for industrial heat need to be scaled up. Additionally, there’s a need for widespread charging infrastructure to support electric vehicles, especially in regions where adoption is still slow. A major barrier is also social resistance: many people are attached to internal combustion engine (ICE) vehicles and hesitant to switch to battery electric vehicles (EVs). Expanding the charging network and increasing consumer awareness are key to overcoming this challenge.
What about heavy industry? Many claim it’s a “hard-to-abate” sector.
That’s a misconception. High-temperature industrial processes can be electrified using resistance furnaces, arc furnaces, inductive heating, and dielectric heaters. Fire bricks, which can store heat up to 2,000°C, are an inexpensive, long-lasting solution. These bricks, made from natural materials, can store excess electricity from renewable sources and release heat when needed, making them an ideal replacement for fossil fuel-based furnaces. They cost about one-tenth per kilowatt-hour of storage compared with batteries and can last indefinitely without degradation.
Regarding emissions from cement and steel production that arise from chemical reactions rather than heat, there are effective solutions. For steel, using green hydrogen instead of coal prevents CO2 process emissions entirely. In cement production, a key innovation is replacing calcite (CaCO3) with basalt, which provides the necessary calcium without off-gassing CO2. Additionally, geopolymer cement is another promising alternative that can eliminate emissions altogether.
Hydrogen is often presented as a key solution for decarbonization. What role does it really play?
Hydrogen is useful, but its role should be limited to specific applications where direct electrification is not feasible. The most suitable uses include green steel production, ammonia synthesis, long-distance transport (especially for long-haul aircraft and ships), and backup electricity storage in select cases. However, hydrogen is not an efficient solution for most transport and heating applications.
For road transport, hydrogen fuel cell passenger vehicles (FCEVs) are significantly less efficient than battery-electric vehicles (BEVs). Producing, compressing, and transporting hydrogen and converting it back to electricity requires much more energy than directly using electricity in batteries. A hydrogen passenger vehicle requires about three to four times the amount of renewable energy to drive the same distance as a battery-electric passenger vehicle. Additionally, FCEVs rely on a complex and costly infrastructure for hydrogen production and refueling, making them less economically competitive than the rapidly expanding EV charging network.
Hydrogen also presents storage and leakage challenges. It is a small molecule that leaks easily from pipelines, leading to efficiency losses and potential safety risks. When blended into fossil gas pipelines for home heating, leakage rates can be significantly higher than for fossil gas, making hydrogen an impractical and inefficient solution for domestic use. Additionally, burning hydrogen in homes or industries releases nitrogen oxides (NOx) and hydrogen oxides (HOx), which contribute to air pollution.
Hydrogen’s role in electricity storage is limited as well. While hydrogen fuel cells can serve as long-term backup, together with existing hydropower and new batteries, in some regions, other regions need only hydropower and batteries, but no hydrogen. In most locations, green hydrogen should be reserved for steel and ammonia production and long-distance transport, but not for building heat, industrial heat, or passenger vehicles.
Some countries, including Italy, are reconsidering nuclear power. Why do you argue that nuclear is unnecessary?
Nuclear is too slow and expensive. Small modular reactors (SMRs) are not commercially viable today, and large reactors take 17-23 years to build in Europe. Even in China, the world’s fastest nuclear developer, nuclear capacity added in 2024 was only 3.9 GW, compared with 370 GW of wind, solar, plus hydro.
SMRs, often promoted as a faster and cheaper solution, face many of the same issues as traditional reactors: high costs, lengthy regulatory approvals, and unresolved waste disposal challenges. No SMR has been built at commercial scale, and even if approved today, it would take at least a decade to bring them online.
Another major issue is that nuclear is not carbon-free. The entire nuclear lifecycle—mining, refining, construction, and waste management—produces emissions. The carbon dioxide equivalent emissions associated with building nuclear reactors are nine to thirty-seven times higher than those from wind energy per kilowatt-hour of electricity generated. Half of this impact is due to the lengthy construction time, which can take seven to ten years, during which significant CO2 emissions are emitted from the background grid. The other half comes from the actual construction of the reactors and from the energy to mine and refine uranium, needed continuously during the life of the nuclear plant. In the case of the Vogel reactors in Georgia, the emissions during construction were enough to build a sidewalk stretching from Miami to Seattle, diagonally across the entire United States.
Wind and solar farms, by contrast, can be deployed much faster and at a fraction of the cost.
Without nuclear, how do you ensure grid stability?
The goal isn’t to provide baseload power but to match supply with demand. Wind and solar can be balanced with batteries, hydropower, and pumped hydropower as well as shifting the times of demand to meet supply (demand response management). Geographic diversification helps smooth variability—for example, interconnecting wind farms over a large area makes wind more stable.
Wind becomes partially baseload when you interconnect wind over large geographic regions. We did a study back in 2007 and found that by interconnecting geographically dispersed wind farms over around a 400 kilometer region in the Great Plains of the US, you can convert completely intermittent wind into an energy source that has about 33 to 47% the reliability of a coal plant. So wind becomes partially baseload.
Enhanced geothermal is another promising solution, with costs expected to drop to $0,08/kWh by 2027, making it competitive with fossil fuels ($0,1/kWh). It taps into heat deep within the Earth, so it’s particularly valuable because it provides constant energy and can be deployed in many locations worldwide.
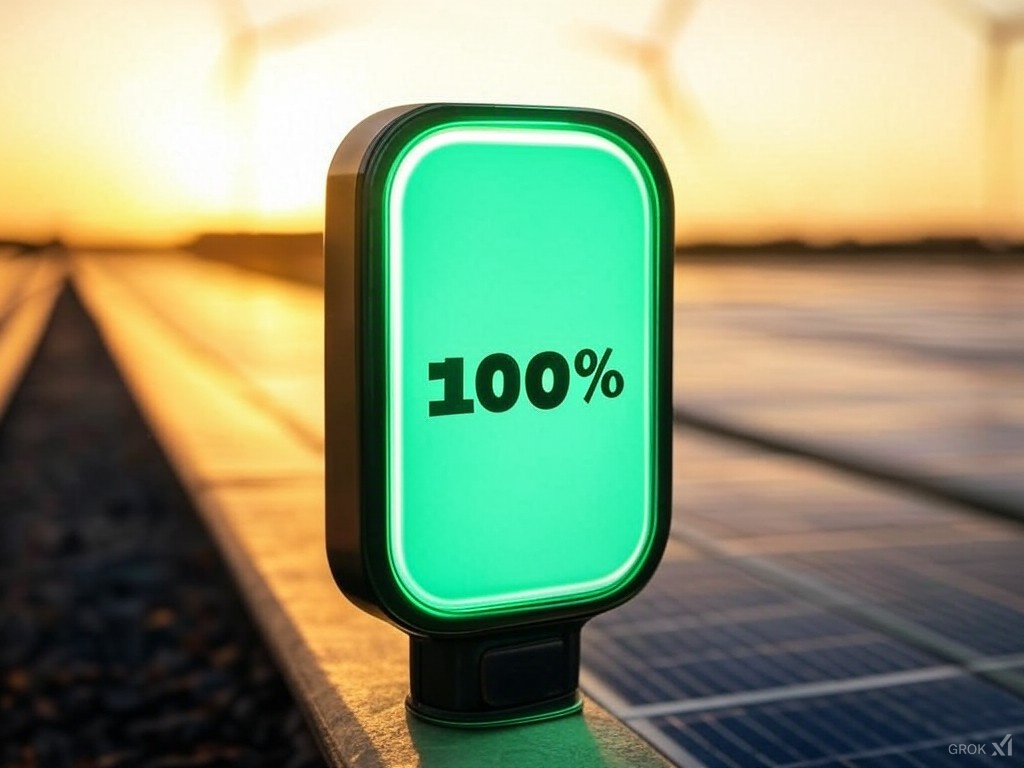
Some worry about the resilience of a fully renewable system. How do we ensure reliability?
The transition happens gradually, with backup systems in place during the process. California’s grid reliability has improved as renewables have increased. Between March and June 2023 versus 2024, for example, solar generation increased by 31%, wind by 8%, and battery storage output doubled. As a result, fossil gas use decreased by 40%, and electricity spot prices fell by 52%, proving that renewables enhance grid stability.
Batteries respond in 20 milliseconds, far outperforming gas plants, which take 300 seconds to ramp up. So gas is actually slower to meet the demand. And you basically need to keep it running and polluting to make sure it’s available. Whereas batteries, you can just turn on and off.
Could trade wars between the U.S. and China slow the transition?
If we achieve 100% wind, water, and solar, energy independence eliminates vulnerability to tariffs. Right now, tariffs on Mexico could immediately raise U.S. gasoline prices, but with an all-electric fleet, such volatility disappears.
Transitioning to renewables improves energy security, lowers costs, and creates jobs—it’s a win-win scenario. Protectionist policies could slow down the transition by increasing the cost of key technologies, but long-term trends favor clean energy expansion.
Moving to policy, what do you expect for the Inflation Reduction Act (IRA) under Trump?
It’s unclear. Trump didn’t repeal existing solar and wind tax credits during his first term, and many Republican-led states benefit from the IRA. Since Republicans only have a slim majority in Congress, major changes may be difficult. However, if the IRA were repealed, clean energy investments would likely continue at the state level, as they did during Trump’s first term.
The IRA has already spurred investment in new manufacturing facilities and job creation. The solar and wind industries are major employers, and many states would resist policies that threaten these growing sectors. It remains to be seen how much a potential rollback could slow down progress.
Is climate diplomacy viable without U.S. leadership?
Yes. The world is moving forward regardless. China installed 370 GW of renewables in 2024, dwarfing the U.S. at around 40 GW. Even within the U.S., states like South Dakota (110% renewables relative to demand) and Iowa (80%) have transitioned due to economic incentives rather than federal policy. The energy transition is happening with or without federal action.
International cooperation remains crucial, but many countries are recognizing the economic and security benefits of renewable energy, independent of U.S. policies. The global shift toward renewables is driven by cost reductions and the urgent need to address climate change.
Some argue Europe has moved too fast with its energy transition, harming industrial competitiveness. Do you agree?
No. China’s transition is much faster—it added 3.5% renewable capacity in one year, yet it’s still on track for 100% clean, renewable energy across all energy sectors (if it electrifies all combustion) by 2046. Most countries are moving too slowly, not too fast. A 5% annual growth rate per year would be ideal.
Concerns about industrial competitiveness should be addressed through targeted policies, such as subsidies for clean technology and stronger supply chain development for renewables. The cost of inaction—rising climate damage, energy insecurity, and missed economic opportunities—far outweighs the short-term challenges of rapid decarbonization.